Chaudhari Lab
Tick and Insect Physiology
Assistant Professor, UNMC Department of Pathology, Microbiology and Immunology
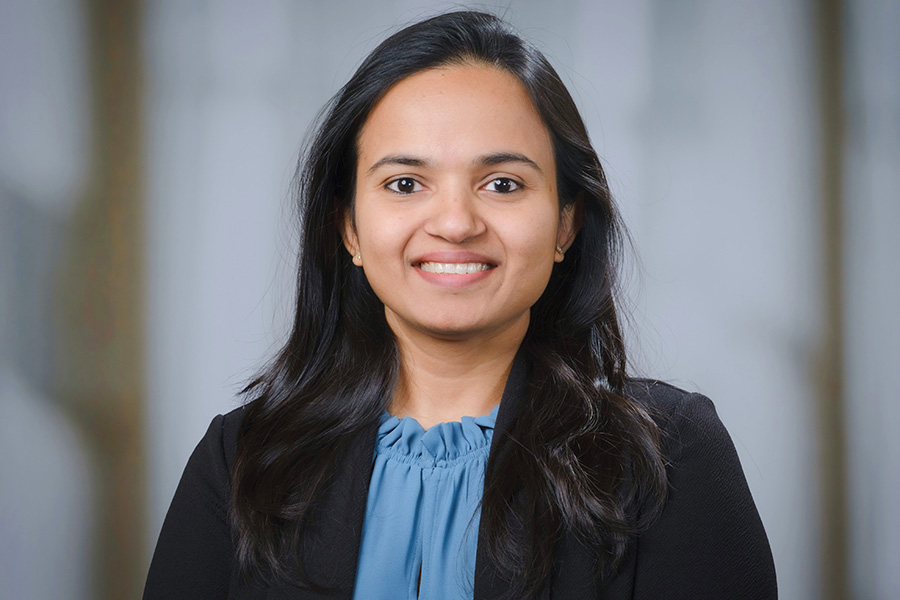
Research in the laboratory of Sujata Chaudhari, PhD, employs cutting-edge molecular biology, genetic, biochemical, and microscopic techniques to study the fundamental developmental processes required for the survival and transmission of pathogens by these vectors. The ultimate objective is to identify new targets in arthropod vectors that can be leveraged to control the acquisition and transmission of pathogens.
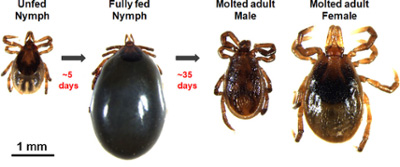
Fig 1. Ixodes scapularis nymphs feed on artificial membranes to complete engorgement in ~ 5 days and molt to the adult stage of development in ~ 35 days
Tick cuticle physiology
Arthropods, including ticks, can transmit diseases to humans as vectors of bacterial, viral, and parasitic pathogens. Ticks are the leading cause of vector-borne diseases in the US, accounting for 95% of reported vector-borne diseases. Borrelia burgdorferi, the causative agent of Lyme disease, is transmitted to vertebrates through the bites of Ixodes scapularis ticks.
I. scapularis ticks feed on mammalian hosts for an extended period of 8-10 days and consume significant amounts of blood, which is crucial for the survival of the ticks as it plays a vital role in their physiological processes such as molting, metamorphosis, and embryogenesis. However, this extended feeding period also puts the mammalian host at risk for acquiring and transmitting pathogens like Borrelia burgdorferi, which can be transmitted to the host through tick saliva during blood feeding.
Once ingested by the tick, B. burgdorferi survives and colonizes in the tick midgut, then migrates from the midgut to reach the salivary glands and is transmitted to the vertebrate host along with tick saliva during blood-feeding. Therefore, strategies aimed at reducing tick blood ingestion could help control the acquisition and transmission of such pathogens.
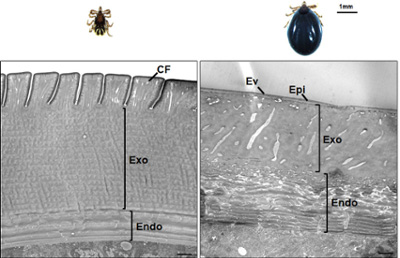
Fig 2. Ultrastructure of Ixodes scapularis unfed fed (left) and fully engorged (right) nymph abdominal cuticle.
The blood-feeding process of Ixodes scapularis ticks involves a significant expansion of their body due to the ingestion of blood. This expansion is made possible by the tick's highly extensible exoskeleton, known as the cuticle, but the molecular mechanisms behind cuticle stretching and its hormonal control have not been well studied. Our work highlights the ultrastructural changes encountered in the tick abdominal cuticle during feeding (Fig 2). As tick feeding is directly dependent on the expansion of the tick cuticle, studying these mechanisms could lead to the development of new strategies for controlling the transmission of pathogens by ticks.
Our research aims to address the following questions related to tick cuticle physiology:
- What molecular mechanisms allow for the expansion of the tick cuticle during blood feeding?
- Is chitin biosynthesis biphasic in ticks, that is, do ticks synthesize and deposit chitin in the cuticle during both blood feeding and molting?
- How is cuticle biogenesis controlled in ticks?
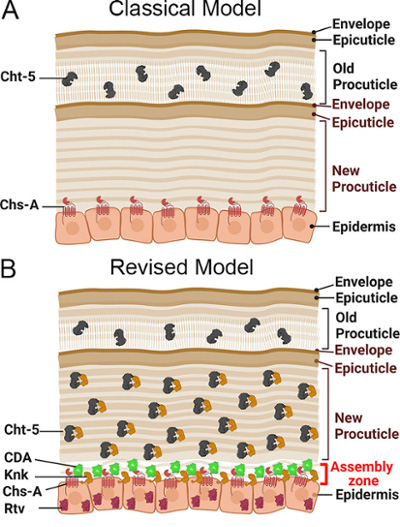
Fig 3. Representation of the insect cuticle during the process of molting.
Insect Physiology
The red flour beetle, Tribolium castaneum, is used as a genetic model to study multiplephysiological processes in insects such as molting, metamorphosis, and embryogenesis. Molting, which involves the replacement of the old exoskeleton with a new one, requires the activity of chitinases to degrade the old cuticle and recycle the resulting products for new cuticle synthesis (Fig 3).
Our paradigm-shifting work has revealed the presence of chitinases in both the old cuticle and the newly developing procuticle. It also demonstrated that the Tribolium castaneum gene knickkopf encodes a chitin-binding protein that co-localizes to the new cuticle and protects chitin from chitinase activity and that another protein called retroactive plays a role in the proper trafficking of Knickkopf to the new cuticle.
We have identified multiple proteins, such as chitin synthases, chitinases, Knk, and Rtv, that play a role in the insect molting process. However, further investigation is needed to uncover other key players involved. Chitin deacetylase is a crucial enzyme known to function during molting.Chitin deacetylase converts chitin to chitosan by the process of deacetylation. Knockdown of the Chitin deacetylase gene results in severe molting defects and high mortality at all stages of Tribolium development. Despite its importance, the mechanism by which Chitin deacetylase regulates molting remains unclear.
Our laboratory employs genetic, biochemical, and microscopic techniques to shed light on the function of Chitin deacetylase enzymes during the molting process.